New frontiers #1
3D Genome
Autoimmunity
Nanopores
Mass spectrometry
Depression
Aging biomarkers
Cryo-EM
Genome engineering
Global medicine
Drugging non-coding targets
If you’re a founder or an inventor in this field, Axial would be happy to help and possibly invest in the company or key experiments.
Axial invests and partners in early-stage life sciences companies. If you or someone you know has a great idea or company in life sciences, Axial would be excited to get to know you and possibly invest in your vision and company — info@axialsprawl.com
Also, go 49ers today - I’m a Packers fan, but I’m a Kyle Juszczyk fan (49ers fullback). He was a nice guy in college especially during IM games between Leverett and Pfoho. Hopefully their defense can contain the Chiefs’ offense.
As always, any list or group of companies is not comprehensive; some companies are stealth-mode and it’s not appropriate to discuss them publicly and I don’t like spending too much time copying and pasting logos.
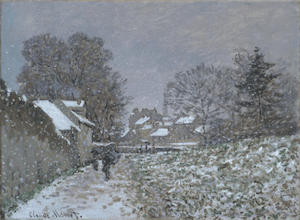
3D Genome
Problem to solve
Understanding the role of the 3D genome in disease. The genome is similar to yarn spooled and re-spooled to have efficient packing - if stretched out, a human genome would measure over 6 feet. Over the last century, the rules of genetics have been established along with characterization of the central dogma. Driven by new tools, the higher-order regulation (i.e. epigenetic) and spatial organization of the genome (3D genome; image below showing the levels of genomic complexity) is being characterized. As the research improves, parts of the 3D genome are becoming attractive therapeutic targets. For example, errors in genome packaging could drive the formation of disease states through various mechanisms. Some of the pathogenic mechanisms include dysregulation of transcriptional and epigenetic changes by chromatin interactions, including dysregulation of transcription, dysregulation of DNA replication, and structural variants and recombination.
How to solve it
There are a set of rules for the 3D genome being characterized that drive the determinism of gene regulation. Genetic sequences loop (146 basepair DNA wrapped around a core histone octamer) and cluster to form topological-associating domains (TAD) where pairwise interactions within a TAD are more likely than outside one. These TADs cluster themselves - with active regions (euchromatin) in the center of the nucleus and inactive regions (heterochromatin) around the periphery. There are more subclasses within the two major regions determined by specific decorations of histone/DNA modifications and complexes. These higher genome structures are incredibly important for health as a little over 1% of the genome encodes proteins (i.e. the genome is not junk). Overall, each level of complexity is regulated by specific markers
New tools to measure and augment the 3D Genome will help identify new targets for human disease:
Imaging - fluorescence in situ hybridization (FISH) and live-cell imaging
Chromosome capture to measure contact probabilities between genomic regions - chromosome conformation capture (3C), circular chromosome conformation capture (4C), chromosome conformation capture carbon copy (5C), chromatin interaction analysis paired-end tag (ChIA PET), and genome-wide chromosome conformation capture (HiC) - have carried most of the freight in the field as sequencing costs have decreased super-exponentially
With applications for:
The use of single-cell sequencing to understand the diversity of 3D genomes within a tissue
The use of super-resolution microscopy to gain a much higher resolution inside living cells
The use of CRISPR to study specific genomic regions in the context of the 3D genome and functionally characterize chromatin interactions
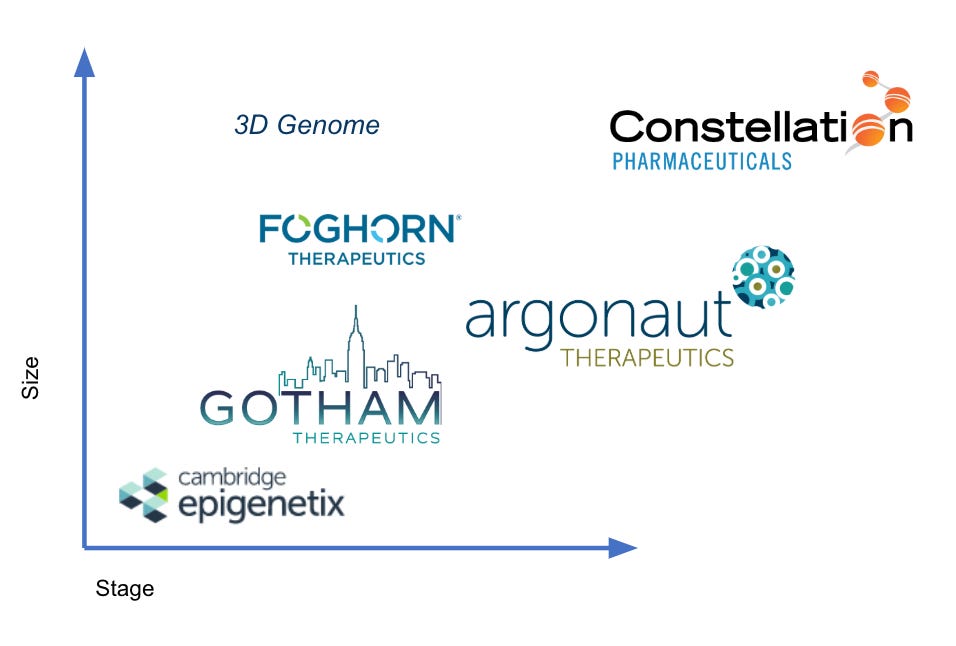
Autoimmunity
Problem to solve
Autoimmune disease affects approximately 5-8% of the world population especially young and middle-aged women. I’ve had a family friend get diagnosed with lupus and another with MS. Autoimmunity is a horrific disease and a large opportunity for drug companies. Autoimmunity is a broad term for ~100 various disease subtypes. All-in-all, autoimmunity can be characterized by excessive and prolonged activation of immune cells.
Most of the disease subtypes are characterized by a focused attack on a particular organ system:
In multiple sclerosis, the brain and spinal cord become inflamed
In both Crohn’s disease and ulcerative colitis, the intestines are damaged
In psoriasis, the skin is the victim
In rheumatoid arthritis, ones joints become inflamed
How to solve it
Autoimmunity is a large problem - hundreds of millions of people and market sizes in the tens of billions. So far, the cutting edge treatment suffers from a hammer looking for a nail syndrome - Humira. Transformative therapies pursuing new targets or using new modalities are needed. An interesting premise is to identify and target self-antigens in each disease subtype to develop therapies for each disorder. Moreover, management tools like Octave Bioscience can be transformative for patients.
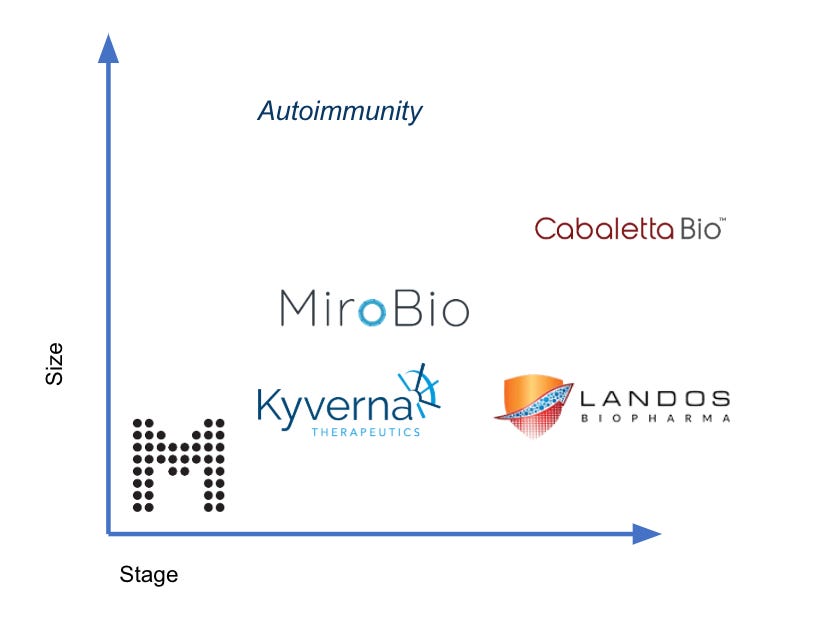
Nanopores
Problem to solve
Sequencing has been a major driver for advances in various fields from clinical diagnostics, synthetic biology, and drug development. The decreasing costs of sequencing is reducing the friction for inventors and companies to just do the experiment. Versus 10-20 years ago, an NGS run always used last when compared to comparable methods like PCR. Increasingly, individual just send their samples off to a sequencing producing vast amounts of data and increasing productivity. To maintain the super exponential decrease in the cost of sequencing, new methodologies need to emerge. Either to change the technical landscape of the field or push incumbents to move forward. With its monopoly position, Illumina does not have the strong incentive to decrease the cost of sequencing dramatically. Twist is in a similar position for synthesis. Nanopores seem to be the best shot to do this for sequencing.
These cost curves going down (i.e. Moore’s Law) are actually economic laws not technical ones. So riding the curves down is finding a balance between measuring demand and making sure the technology can meet it. For sequencing, demand is red hot in some places (i.e. research, parts of drug development) and not so much in others (i.e. clinical diagnostics); however, a stepwise decrease in costs ought to expand the former and ignite the latter.
How to solve it
There are three major types of nanopores:
Biological – high fidelity, low throughput
Solid state – low-to-medium fidelity, high throughput
Mechanical – high fidelity, low-to-medium throughput
Some variant of a nanopore will need to have the proper coverage, cost, speed, and error rate combination to be useful in various markets: drug development, diagnostics, synthetic biology.
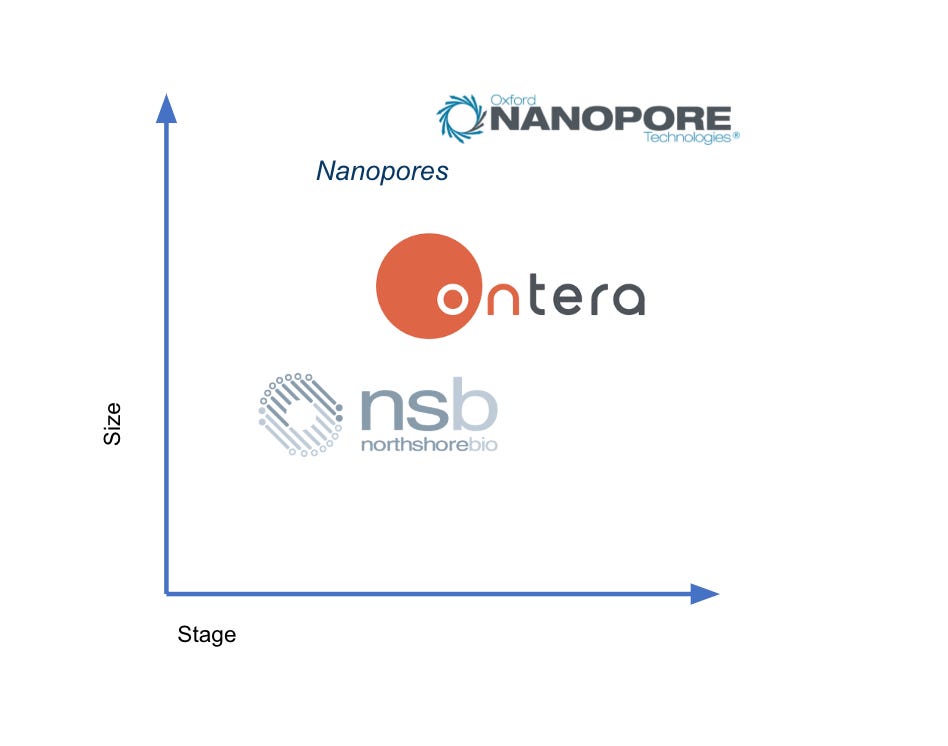
Mass spectrometry
Problem to solve
A bottleneck for the expansion of proteomics for drug development and diagnosis is the accuracy and somewhat the cost of mass spectrometry. Ultimately, to make mass spec clinical-grade, these two issues need to be addressed. The potential is vast: expanding the use of metabolomics and proteomics for clinical use, the development of new protein biomarkers, and so much more. Actually, nanopores might play a role here.
How to solve it
Mass spectrometry enables direct identification of molecules based on the mass-to-charge ratios as well as fragmentation patterns. Simply, two problems need to be optimized:
Develop better restriction enzymes to improve fragmentation
Improve algorithms to improve signal-to-noise ratio
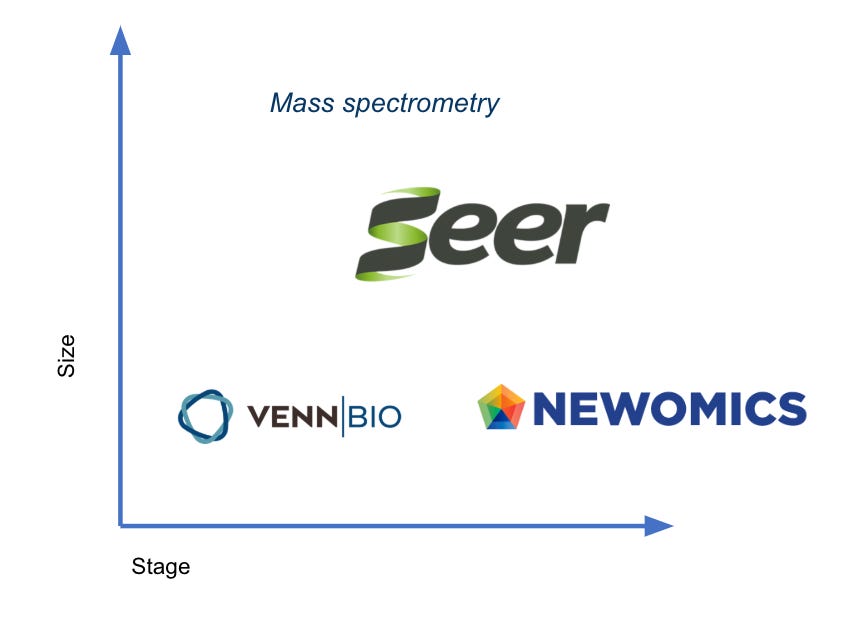
Depression
Problem to solve
Depression might be the most neglected disease - it’s hard to measure and it’s taboo to either tell someone or accept it. The next 10 years for individuals with depression with regards to treatment looks pretty dim. There are only a handful of drug companies truly pursuing ambitious programs, mental health payments within our healthcare system is overly complex, and the features to measure/augment are not fully characterized.
How to solve it
To make a dent in depression, better diagnosis and treatments need to emerge. The payment side is a little trickier and might be pushed a bit by the technology and more-and-more awareness. Say for cancer, the diseases can be effectively treated with an antibody or cell therapy. Cancers and their mechanisms are pretty well understood. Depression is not. It’s a complex phenotype seemingly with a lot of variation. As a result, both molecular and digital therapeutics might have equal chances are improving the lives of depression patients. In addition, new biomarkers to treat depression would be useful. Also, medical devices and prosthetics could also play a role to treat depression.
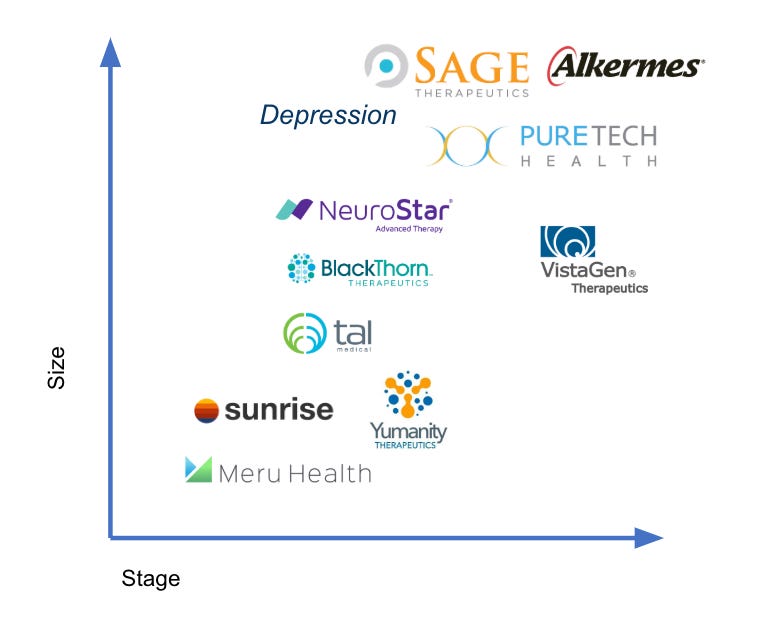
Aging biomarkers
Problem to solve
Aging is a large problem for society. However, most companies in the field just don’t know what to measure. As a result, more of the capital being put into the field probably will go to waste. Many longevity companies are hindered by the inability to define the parameters of aging itself. Even if biomarkers of aging are validated, determining which biomarkers to measure in trials of each drug is the next challenge.
How to solve it
On the basis of the current literature, many physiological parameters that are indicators of frailty in humans might serve as readouts for aging. These include, but are not limited to, metabolic health, cardiac function, blood pressure, bone health, body weight, muscle strength, cognitive performance, cerebral blood flow, brain atrophy, circulating hormone levels, cataract formation, immune response, and tumorigenesis. With the existing set of tools available, companies measuring some of these features and figuring out how to translate them into biomarkers and their corresponding drug are probably solving the most important problem in aging.
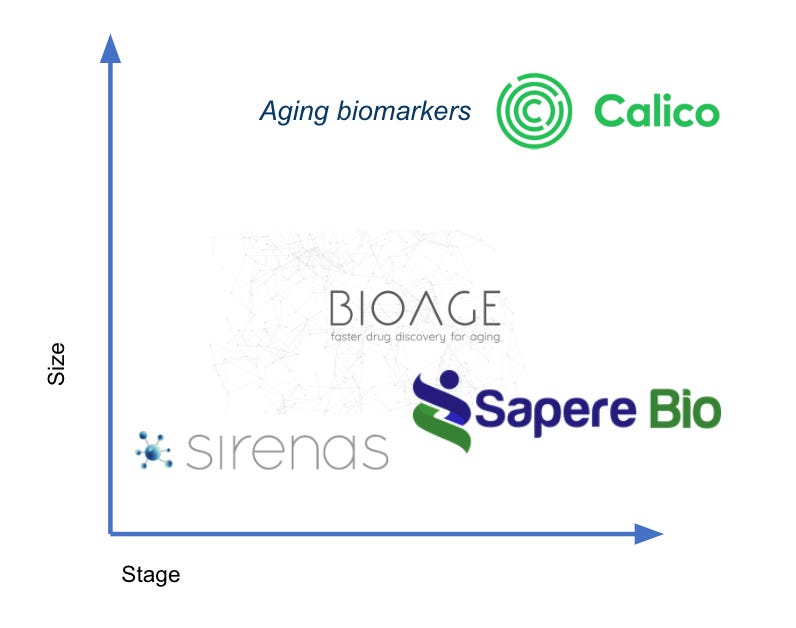
Cryo-EM
Problem to solve
There is a revolution going on with cryo-electron microscopy (cryo-EM) to capture dynamic protein structures. In the early 2000s there was only a handful of protein structures derived from cryo-EM (~10). Now at least a thousand structures have been solved with the tool and this number is growing rapidly. This has been driven by the increasing resolution of images being produced by cryo-EM. The number of new structures being discovered using cryo-EM as well as various configurations of these structures is growing rapidly and surpassing that of more traditional methods like X-ray crystallography. Moreover, there are improvements in other biochemical and biophysical methods as well (i.e. atomic force microscopy). With the toolkit of determine molecular structure and motion rapidly improving, there is the potential to create a biochemical and biophysical assembly line to discover better medicines.
How to solve it
Cryo-EM uses a beam of electrons to map the structures of proteins and other biomolecules. Cryo-EM is particular useful to determine the structural characteristics of membrane proteins and other molecular targets that have been intractable to other methods such as X-ray crystallography and NMR. A few bottlenecks need to be solved before more companies can emerge centered around cryo-EM:
The first limitation is the actual cost of a cryo-EM device - about $10M. Figuring out how to either decrease the cost per device or figure out a way to maximize access and use of existing ones would be useful.
The most important problem is processing the large amounts of data produced. One microscope can produce 1-2 TB of data per day. In practice, most of this data is left unprocessed. Better software will be a key driven to ingest this growing data set and set up drug discoverers to develop better medicines.
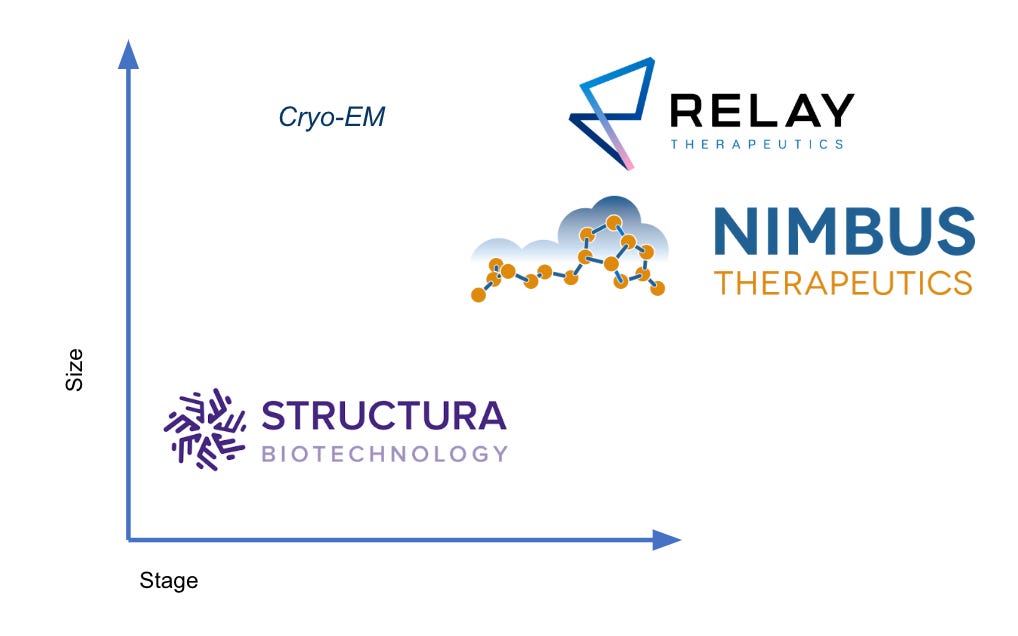
Genome engineering
Problem to solve
The last two decades of synthetic biology has enabled the design and production of new organisms with completely unique genomes. Many parts of science-fiction from a century ago are actually possible now. The commercialization part has been the difficult part - what do customers want? At what price? Scaling up genome engineering (i.e. editing at thousands of sites) to create more organism variants can create a new paradigm for drug discovery, entirely new consumer experiences, and replace important parts in industrial/manufacturing processes.
How to solve it
Despite the enormity of the opportunity, there are some pretty substantial technical challenges within genome engineering. There are only a handful of labs with the proficiency to truly make genome engineering useful. There are two major methods right now:
Multiplex automated genome engineering (MAGE) - targets multiple locations at the same time within one cell or across many
Conjugative Assembly Genome Engineering (CAGE) - large-scale assembly of a genome while programming specific modifications
For business, the most exciting opportunity is the ability for these tools to create new models to screen for new medicines. Genome engineering could scale up models Blueprint and Loxo have laid out.
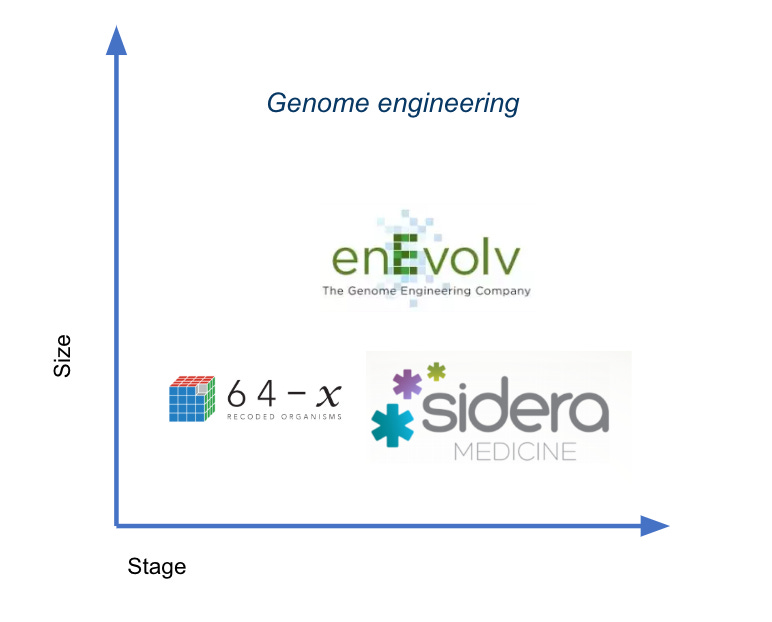
Global medicine
Problem to solve
Global medicine is an idiosyncratic opportunity but potentially can transform the entire industry of clinical development and healthcare. Most trials for new medicines occur in the US. Limiting companies to a subset of patients creates issues with recruitment and diversity. Restricting care to one geography keeps costs high (i.e. globalization has export a lot of jobs out of the US, but hasn’t really affected healthcare) and reduces patient choice.
How to solve it
Despite the potential here, not that many companies are focused on the problem. Changes in laws that would let patients easily get care in any country. This is probably unlikely given the monopoly power of the AMA. However, the ability for global medicine to improve the development of new drugs, diagnostic tests, and a wide range of life sciences products is possible. Companies and products around a set of tasks could make it easier for companies to find patients and make new products:
Interoperable clinical trials - solving patient recruitment, matching, and retention
Logistics tracking and privacy of patient samples
Deployable infrastructure (i.e. clinics, healthcare practitioners) to specific populations
Data analysis across large cohorts of patients from different backgrounds (i.e. use of master protocols and basket trials)
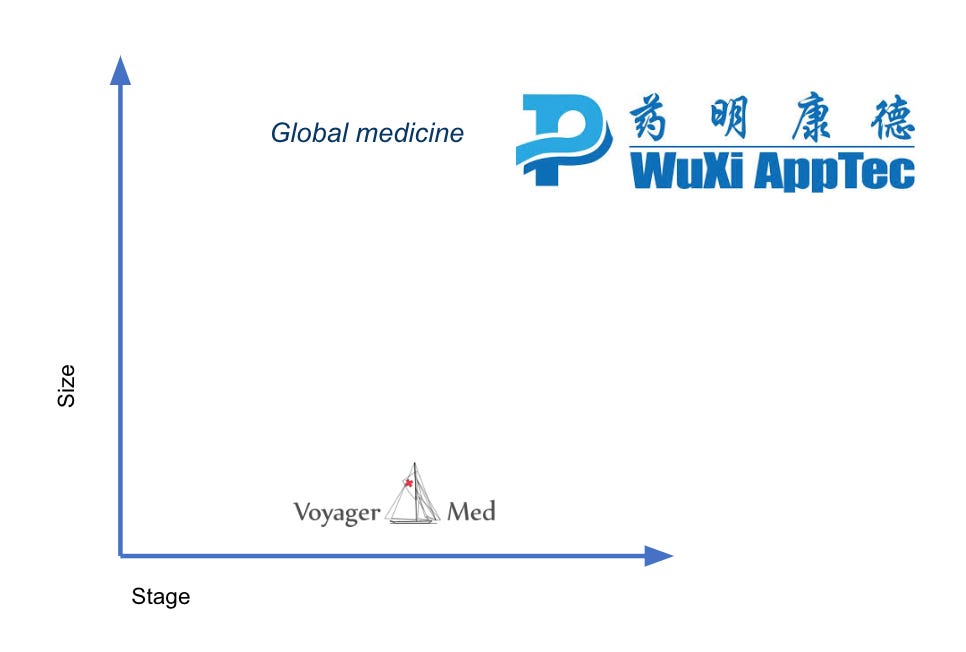
Drugging non-coding targets
Problem to solve
The discovery of tens of thousands of noncoding RNAs (ncRNAs) has opened them up for targeting by drugs. Noncoding RNAs represent ~70% of the genome and only 1–2% encode for proteins. However, many noncoding RNAs have proven to be undruggable.
How to solve it
There have been a lot of false starts in the field. Ten years ago quite a few companies emerged to solve this problem, but were a few decades out from the clinic. With progress across the field of the non-coding genome, the potential of companies centered around ncRNAs is actually pretty exciting given the new biology and shorter window into humans (versus a decade ago; time solves a lot of problems). Two major issues that need to be improved upon are:
Getting a better understanding of RNA – form and function
The floppy RNA model is not entirely accurate – portions of some RNAs are actually rich in structure, and pinpointing these regions will make it easier to tackle with small molecules
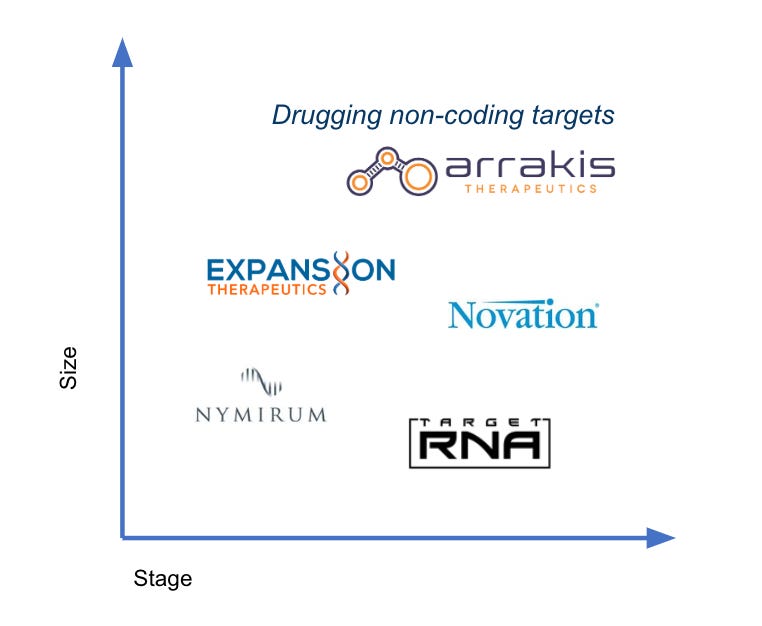